Good news - we're living in a golden age of black hole discoveries
Toggle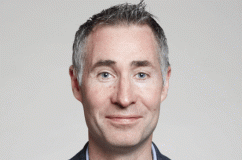
Black holes are at once both extremely simple and incredibly complex. In simple terms they are defined by only their mass, their charge and their spin. In our Universe, at least, black holes are expected to be chargeless and we won’t worry about a black hole’s spin here either, so the most important characteristic of a black hole is simply its mass.
Our observations to date show that black holes come in two flavours. On the one hand we have stellar mass black holes: these are black holes that are between about 10 and 200 times the mass of the Sun. These objects form after massive stars end their lives and succumb to the overwhelming force of gravity. Once a massive star stops burning it can no longer support its immense weight and inevitably collapses to form a black hole. On the other hand, we also observe extremely large black holes with masses in excess of one million times the mass of the Sun, dubbed super-massive black holes. These behemoths are so super-massive and have such a huge effect on their host galaxy it allows us to "see" them. The trouble is we don’t know where the monster black holes came from - we don’t know where, when or how they started life!
We can't directly see black holes. Any light straying near to black holes gets swallowed up and can never escape (hence the name). Nothing can escape a black hole and hence we could never, even in the far future, send a probe into a black hole as we would never get any information back again. However, we can detect them indirectly. One of the most amazing events of the last decade across all of science has been the detection of Gravitational Waves. Twenty five years in the making, two detectors in the US, one in Washington state and the other in Louisiana, detected the first gravitational waves in September 2015. The first event was actually captured during an engineering test cycle, after upgrading the detector, and as such was very unexpected.
In any case the detection was seismic. It proved once and for all that gravitational waves could be detected. It also opened up a completely new window on the Universe. The event itself, of course, was mind blowing. The two black holes that merged had masses of 39 and 36 times the mass of the Sun respectively. The merger instantly converted three solar masses into energy - more precisely into gravitational waves. To put this number in context - your normal household nuclear warhead converts about 1 kg of nuclear material into energy. The merger of two black holes converted 1030 kgs of material (that’s a ten followed by 30 zeros) into gravitational waves.
These gravitational waves then travel through space, at the speed of light, making their way to us here on Earth. However, the detection itself is an immense challenge. Space is incredibly tough and gravitational waves work by bending and warping space-time and that signal propagates through space to us. You can think of this as being like shaking a long rope and watching the wave propagate along the rope. Gravitational waves compress and stretch space in much the same way. But like I said, space is tough. Even the conversion of three solar masses into pure energy doesn’t bend space that much. What LIGO (Laser Interferometer Gravitational Wave Observatory) detected was the stretching of space-time by the width of a human hair. Yes that’s right: the distance from Earth to the next nearest galaxy (a few million light years away) got stretched by the width of human hair.
LIGO managed this incredible feat by firing lasers down two arms positioned at right angles to each other. LIGOs arms are 4km long and it bounces the laser beam down hundreds of times. Any difference then in the return times between the two lasers means that something happened. More often than not any difference is not due to a gravitational wave (usually it’s seismic activity on earth or a large truck going past). But in September 2015, for the first time ever, both detectors detected clear evidence of gravitational waves.
However, LIGO has intrinsic limitations. It can only detect black holes in the relatively nearby Universe and it can only detect black holes merging in a certain mass range (stellar mass black holes). It's not sensitive to the mergers of truly massive black holes we think inhabit galactic centres and it can’t 'see’ out to very large distances. However, plans are afoot to go searching for their more massive cousins - black holes with masses between 10,000 and 10 million times the mass of the Sun.
LISA - Laser Interferometer Space Antenna - has been on the drawing board for well over two decades. The detection of gravitational waves by LIGO provided the catalyst for ESA to formally adopt the LISA mission in December 2023. This was a seminal moment for myself and other black hole astrophysicists who have been studying these massive objects all our careers. LISA will work in space. Instead of arms that are 4km long, as LIGO has, LISA will have arm lengths of 2.5 million kilometres. With such long arms LISA will be able to detect the mergers of massive black holes across the entire observable Universe. It will fly in an orbit behind the Earth and so will orbit the Sun just as the Earth does. Due for launch in 2034 (which in cosmological terms is equivalent to a very short nap), astrophysicists the world over are extremely busy positioning themselves to make best use of the data. In fact, this summer Ireland will host the LISA Symposium, where physicists across multiple domains will come to discuss the LISA mission.
It feels like we are currently living in a golden age of black hole discoveries but I have a very strong feeling that we ain’t seen nothing yet.
This piece originally appeared on RTÉ Brainstorm